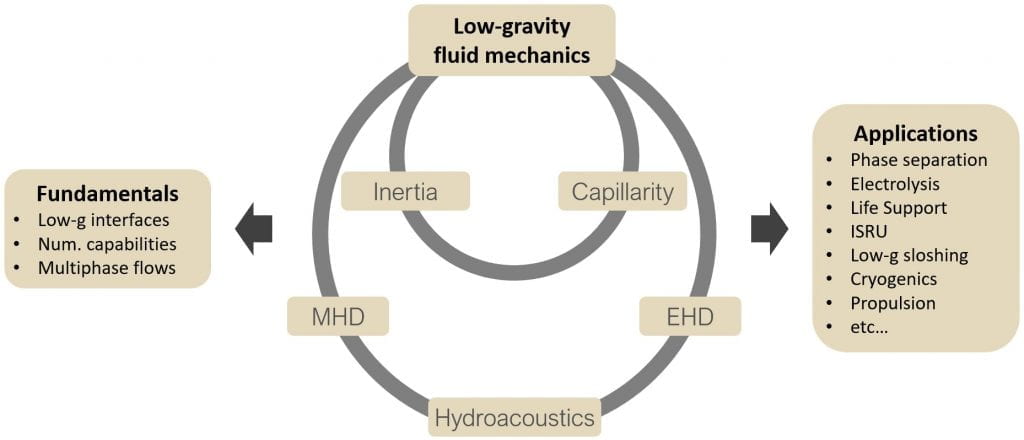
Reliable and efficient multiphase flow management technologies will become increasingly relevant for the development of space systems as commercial space stations start operating at LEO and humans venture once again into outer space. The goal of the Low-Gravity Science and Technology (LGST) Laboratory is to face the many challenges that this transition will involve by expanding the definition of low-gravity fluid mechanics, traditionally limited to the combination of inertial and capillary forces, to include magnetohydrodynamic (MHD), electrohydrodynamic (EHD), and hydroacoustic (HA) actuation mechanisms. Our ultimate goal is to master these and additional unexplored physical mechanisms to develop the next generation of space systems.
Dia/Paramagnetic Phase Separation

Phase separation in microgravity is crucial for a wide variety of spacecraft processes, such as propellant management, heat transfer, oxygen and fuel production, and the reduction of carbon dioxide, among many others. The list of phase separation methods is vast and includes centrifuges, forced vortical flows, wedge geometries, or thruster settling. Different research groups have also explored the use of electrohydrodynamic or hydroacoustic approaches with different degrees of success, but so far, these ideas remain in a very early stage of development.
Complementary to the aforementioned methods, the inherent dia- and paramagnetic properties of liquids can also be employed for passive phase separation. We have just presented our first validation experiments in microgravity in a recent journal article at npj Microgravity and are now proceeding to apply this patented technology to the development of a wide range of space systems.
Magnetically-Enhanced Electrolysis

Keeping astronauts breathing aboard the International Space Station (ISS) and other space vehicles is a complicated and costly process. The Oxygen Generation Assembly at the ISS employs electrolytic cells to split water into oxygen and hydrogen. However, centrifuges with large mass, power, and maintenance penalties are currently required to get the gases out of the system. A relatively recent analysis from Harry W. Jones at NASA Ames concluded that it would be unfeasible to adapt the same architecture on a trip to Mars. Therefore, a different approach is needed.
The LGST Lab is currently exploring how to apply magnetohydrodynamic forces to split and collect hydrogen and oxygen using a monolithic water-splitting device in microgravity. Our ultimate goal is to eliminate moving parts and enhance the reliability and efficiency of the system by simplifying its architecture. More details can be found in one of our journal articles and our list of patents.
Simulation of coupled fluid-magnetic flows
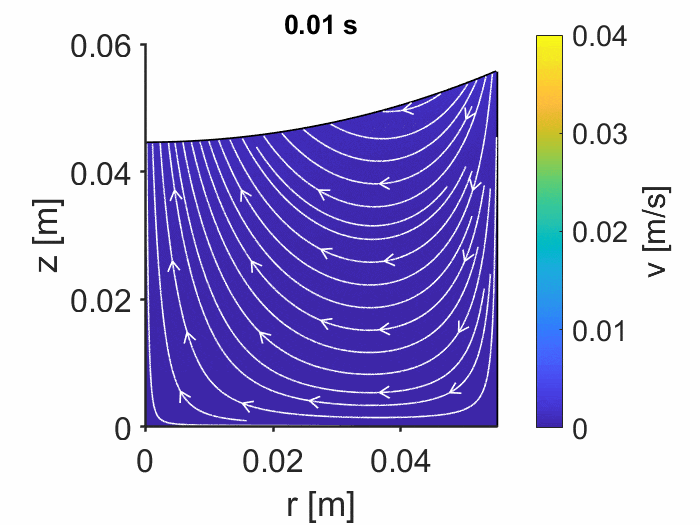
Several systems in physics and engineering employ highly susceptible magnetic liquids to touchlessly control the behavior of multiphase flows. This intersection is particularly prolific in milli/microfluidic and space applications, where surface tension overcomes gravity and only a limited range of actuation mechanisms is available.
Our group has recently introduced and validated the very first coupled ferrohydrodynamic interface-tracking model for the analysis of the equilibrium, linear stability, and modal response of magnetic liquid interfaces in surface tension-dominated axisymmetric multiphase flows. This, together with our previous analytical works, allows us to study a whole new family of complex multiphysics problems involving ferrofluids. Applications range from active propellant sloshing control to the development of stable liquid mirrors.
Propellant sloshing
The sloshing of liquids entails several technical challenges for spacecraft designers due to its effects on the dynamics and operation of space vehicles. While hypergravity sloshing gives rise to complex fluid-vehicle interactions, in microgravity propellant retention and engine restart become the most significant challenges. Traditional propellant management devices have limited applicability in cryogenics or conformal tank geometries, which are becoming increasingly relevant for on-orbit refueling, in-situ resource utilization, and propelled SmallSats. These applications are in a very early stage of development, and further efforts are required to advance their technology readiness level.
The LGST Lab is exploring a different approach to low-gravity sloshing control based on the imposition of body forces such as paramagnetism, dielectrophoresis, and sound waves. Many of these technologies were proposed in the 1960s, but it is only now that we have missions where they can really have a significant impact. In addition, our lab is recovering and updating the traditional analytical sloshing design methods and creating open-source tools to make them accessible for everyone.